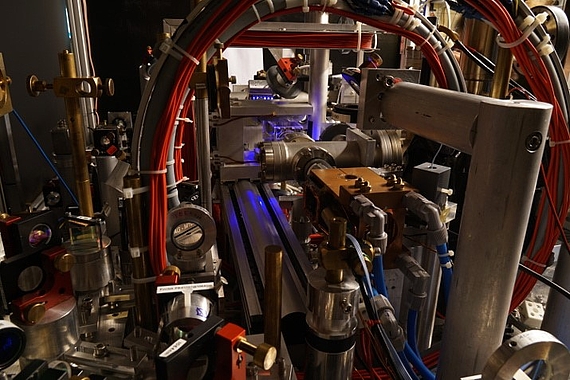
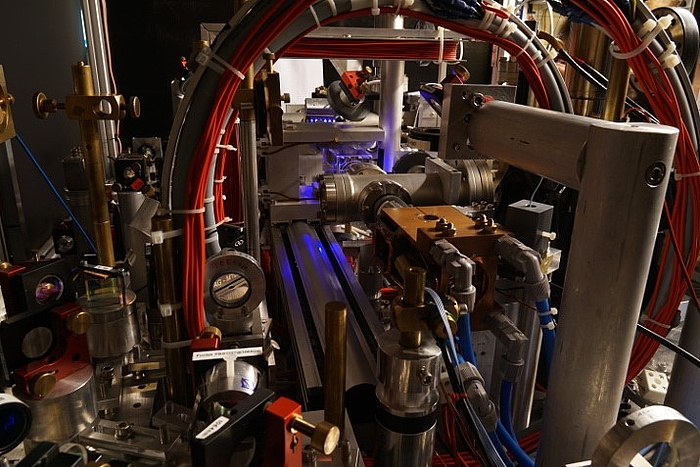
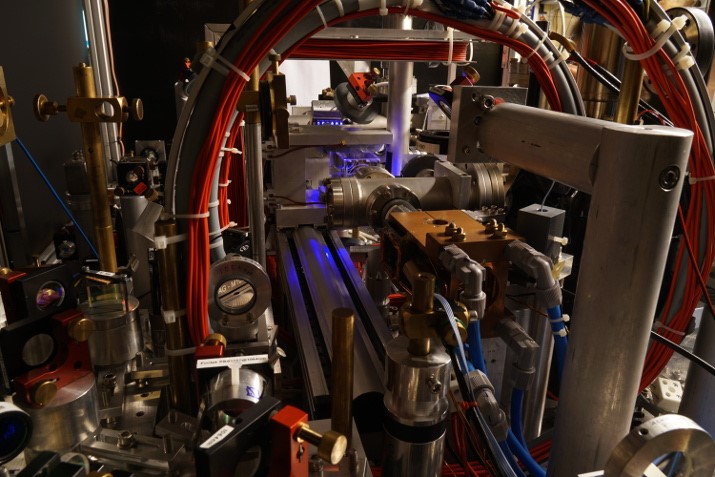
Introduction
In the beginning of the second funding period, the generation of highly entangled states was mainly restricted to the creation of spin correlations, where the external degrees of freedom remain untouched. However, the creation of entanglement in external degrees of freedom is highly desirable: Entangled atomic states in external degrees of freedom can improve inertially sensitive atom interferometers beyond the Standard Quantum Limit (SQL). Entangled ensembles of ultra-cold atoms provide an ideal source for highest-precision atom interferometry, as they offer perfect mode control and low densities to suppress systematic effects, and offer a superior phase resolution for a given atomic flux. Furthermore, they constitute fascinating quantum-mechanical probes for tests of fundamental physics, most prominently in their interaction with gravitational fields.
Results
Our generation of high-fidelity Twin-Fock states enabled sub-SQL interferometry, featuring a high degree of entanglement. However, the employed process led to strong fluctuations of the total number of particles. The fluctuations are unwanted for the application of Twin-Fock states in atom interferometry, where a steady atom number is desirable. Based on our study in the first funding period and previous work, we have implemented the adiabatic generation of entangled states.
We have constructed a Raman laser system, where the laser intensities of the two beams and the relative phase between them is actively stabilized. Because of the intensity stabilisation, the Raman laser can be precisely operated on the specific intensity ratio, where it is insensitive to first-order intensity drifts, enabling an unprecedented contrast close to 100%.
Based on the adiabatic generation of Twin-Fock states and the developed Raman laser system, we were able to create Twin-Fock states in spin space and transfer them to momentum space. This achievement presents an important requirement for entanglement-enhanced gravimetry.
We have used our system of Bose-Einstein condensates with spin degree of freedom for an investigation of excited-state quantum phases, as proposed for the second funding phase. The ground-state phases of a quantum many-body system are characterised by an order parameter, which changes abruptly at quantum phase transitions when an external control parameter is varied. Interestingly, these concepts may be extended to excited states, for which it is possible to define equivalent excited-state quantum phase transitions. However, the experimental mapping of a phase diagram of excited quantum states was not realized so far. We were able to demonstrate the experimental determination of the excited-state phase diagram in our spinor Bose-Einstein condensates, where, crucially, the excitation energy is one of the control parameters.
Publications
Showing results 1 - 13 out of 13
Project leader
30167 Hannover
30167 Hannover